Alternative fuels and clean driving: the foundation for green mobility and successful climate protection
The transport industry has a pivotal role to play when it comes to combatting climate change and safeguarding the environment. As one of Europe’s largest CO2 emitters, the sector has a responsibility to spearhead decarbonization efforts. Currently, approximately 8.1 million trucks weighing 3.5 tons or more are responsible for around one-third of CO2 emissions in road transport.
Governmental directives are also driving the pursuit of low-carbon mobility. To meet Europe’s binding zero-emissions target by 2050, the European Commission (EC) mandates that new lorries must reduce their emissions by 90% by 2040. For 2030, the EC has set a new interim goal of a 45% reduction in CO2 emissions (previously -30%) compared to 2019, with a revised target of 65% by 2035. To accelerate the transition to zero-emission public transport throughout Europe, new city buses must reduce their emissions by 90% from 2030 and all new city buses must be emission-free by 2035. As truck manufacturers, the TRATON GROUP and its Scania, MAN, International and Volkswagen Truck & Bus brands recognize this responsibility and view the adoption of battery-electric trucks and buses as a critical means of achieving a CO2-neutral energy transition in transportation.
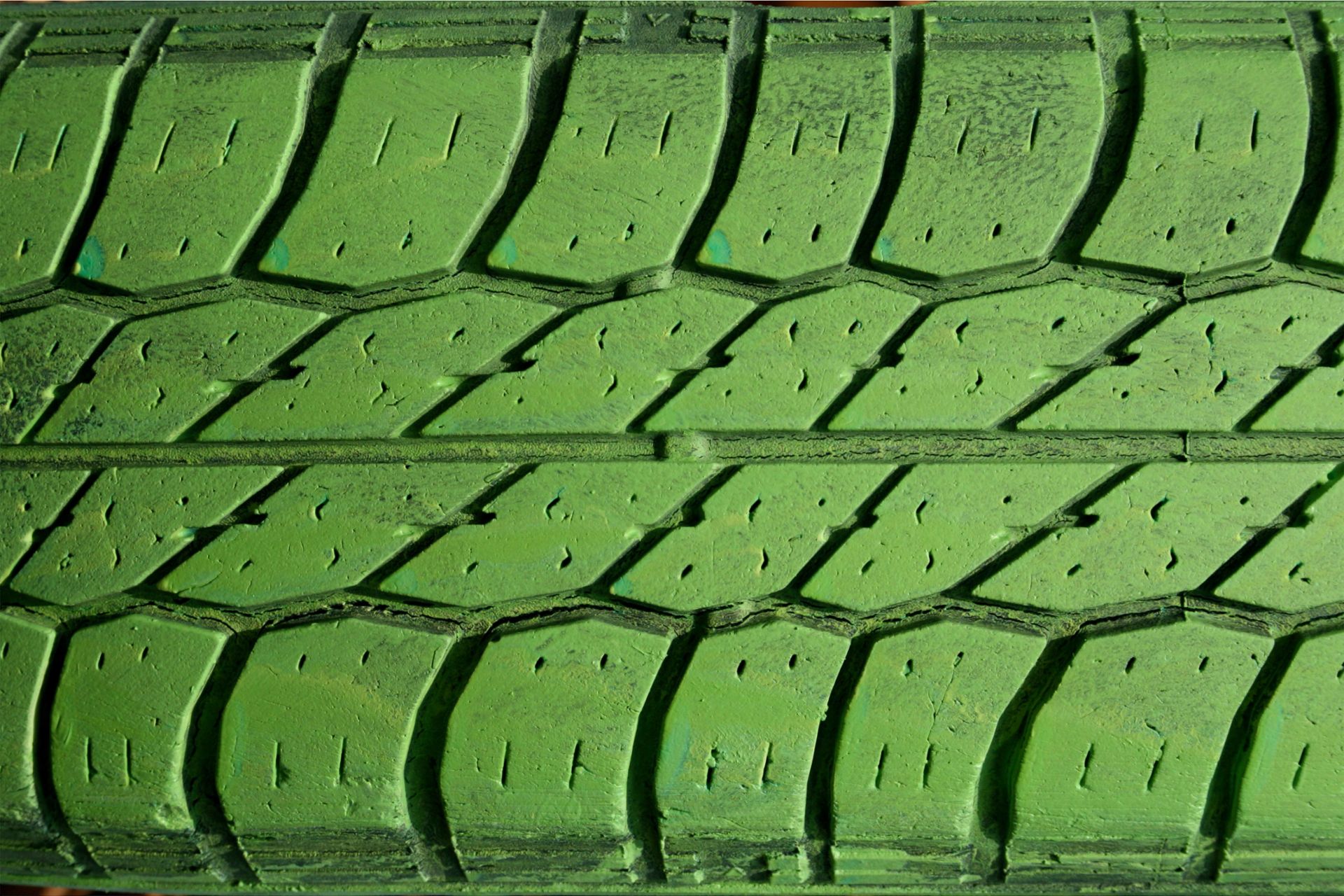
Undoubtedly, electrifying transportation stands as the decisive step in the fight against climate change. Electric buses and trucks, powered by batteries, emit zero carbon dioxide compared to gasoline or hybrid trucks. Therefore, electric buses and trucks make a significant contribution to the reduction of greenhouse gas emissions. For instance, replacing 10,000 diesel trucks in long-distance transport with electric vehicles could save up to one million tons of CO2 annually. This impact is not only substantial, but also readily achievable, given that battery technology, electric motors, and power electronics are already available. However, the electrification of the transport sector poses a multifaceted challenge, especially when it comes to establishing a comprehensive charging infrastructure spanning countries and continents. Developing an efficient charging infrastructure for electric vehicles remains a formidable task for both public and private operators. For example, the EU's Alternative Fuels Infrastructure Regulation (AFIR) mandates comprehensive coverage of charging facilities along European transport routes for battery-electric commercial vehicles by 2030.
However, until widespread adoption of commercial vehicles with battery-electric drivetrains occurs, traditional internal combustion engine vehicles running on diesel will persist. In this interim period, employing CO2-neutral fuels can serve as a pragmatic step toward achieving the zero-emissions target by enabling climate-neutral and clean driving.
Multifaceted and diverse: how alternative fuels facilitate climate-neutral driving
A widely debated aspect of achieving climate-neutral driving and realizing EU targets involves the utilization of so-called clean fuels. These are CO2-neutral liquid or gaseous fuels derived from electricity, ideally obtained from renewable sources such as solar and wind energy. Also known as alternative fuels, renewable fuels, or green fuels, these options boast a high energy density and ease of storage. In addition, no technical adjustments are required for combustion engines when using these fuels. However, the conversion process incurs energy losses, resulting in significantly lower efficiency compared to battery-electric vehicles. Additionally, there is presently a lack of industrial-scale infrastructure for clean fuels. Furthermore, production costs remain prohibitively high.
The following alternatives are regarded as clean fuels:
Hydrogen: The chemical element H2 could play an important role in advancing green mobility. Fuel cells in trucks, for example, utilize hydrogen to generate electrical energy. However, the challenge lies in establishing a nationwide refueling station infrastructure for hydrogen fuel cell vehicles and trucks. Until then, energy-intensive sectors, such as the chemical industry, may rely on hydrogen to meet climate targets, often accessing it more affordably than long-distance transport. For the latter, utilizing renewable electricity directly in battery-electric trucks proves to be more effective and energy-saving. While hydrogen will play a crucial role in the future energy system, its application may vary across sectors. It will likely take decades before green hydrogen is available in sufficient quantities.
E-fuels: Synthetic fuels, ideally obtained from renewable energy sources using the Fischer-Tropsch process, offer potential as sustainable alternatives. This process involves converting a mixture of hydrogen and carbon dioxide into liquid hydrocarbons. However, drawbacks include significant energy loss during conversion and a lack of industrial infrastructure.
Biofuels: This category includes HVO (hydrotreated vegetable oil), biodiesel, bioethanol, biomethane, and biogas. These are produced using organic, predominantly plant-based materials and can be utilized in existing engines. As no additional carbon dioxide is released into the atmosphere during combustion (only the CO2 previously bound by plants and animals is released), these alternative fuels are considered CO2-neutral in balance sheet terms, though not locally emission-free. The use of food crops for HVO, biodiesel and bioethanol remains a subject of controversial public debate. Biomethane and biogas are produced through the fermentation of agricultural waste, sewage sludge, or organic waste.
Facts about clean fuels: production
- Power-to-liquid (PtL) applications play a crucial role in producing clean fuels. Hydrogen, generated from water or desalinated seawater through electrolysis using electrical energy, serves as the foundation for these fuels. Hydrogen can be utilized in its pure liquid or gaseous form.
- Hydrogen serves as the primary ingredient in producing synthetic fuels such as e-diesel through the Fischer-Tropsch process.
- In the production of hydrogenated vegetable oils (HVO), hydrogen facilitates a chemical reaction that removes oxygen from the (old) vegetable fat, resulting in a liquid with a similar chemical composition to fossil diesel.
- Biodiesel (FAME = fatty acid methyl ester) is derived by converting vegetable oils into molecular fatty acid methyl esters with the addition of methanol, a by-product of hydrogen. In the EU, primary raw materials include used cooking oil and rapeseed oil, with lesser amounts of palm oil, sunflower oil, and soya oil. Biodiesel is sensitive to cold temperatures.
- Bioethanol, biomethane, and biogas, do not require catalytic converters. Through fermentation, microorganisms break down agricultural raw materials like grain and sugar beet, and residual materials such as straw (for bioethanol), as well as agricultural waste, sewage sludge or organic waste (for biomethane and biogas). This process produces methane which, like natural gas, can be used to generate electricity, for heating, or as an alternative vehicle fuel.
Facts about clean fuels: use and emissions
Hydrogen: green mobility with fuel cells
Fuel cell commercial vehicles are an alternative to conventional diesel trucks. Fuel cell technology utilizes compressed liquid hydrogen, stored in a tank on-board the hydrogen truck or hydrogen bus, as a fuel source to generate electrical energy. The compressed hydrogen is then directed to the fuel cell within the drivetrain, where it reacts with oxygen from the air and generates electrical energy. This electricity powers an electric motor, which subsequently propels the vehicle’s wheels. Notably, the only byproducts of this process are water and a minimal amount of heat. Consequently, fuel cell drives produce virtually no exhaust gases during combustion, rendering them nearly emission-free.
Hydrogen: a versatile energy carrier with varied origins
While hydrogen itself is colorless, its diverse origins give rise to its association with various colors:
-
Produced through water electrolysis using renewable electricity. This involves a chemical reaction in an electrolyzer to split the water into its individual components of hydrogen and oxygen. The only waste product is oxygen, which is released into the air.
-
Typically derived from fossil gas. One ton of grey hydrogen produces approximately ten tons of CO2, which is released into the atmosphere.
-
This is grey hydrogen with captured and stored carbon dioxide during production, making it almost CO2-neutral.
-
Generated via the thermal decomposition of methane (methane pyrolysis); solid carbon is produced instead of CO2.
-
Produced using electricity from nuclear power. Similarly to the green hydrogen production process, it utilizes electrolysis.
-
Created from biomass energy and electricity from waste-to-energy plants, often involving electrolysis.
-
Naturally occurring hydrogen found underground in deep layers of rock. Can be extracted through methods like fracking.
-
Produced through water electrolysis using a blend of renewable and fossil electricity sources, such as wind or solar power, alongside energy from coal-fired power stations.
-
Black hydrogen: Generated using electricity from hard coal. With the decision to phase out coal in countries like Germany, black hydrogen will be a less viable option in the future.
-
Produced utilizing energy from lignite, another form of coal.
E-fuels – unlocking the potential of synthetic fuels
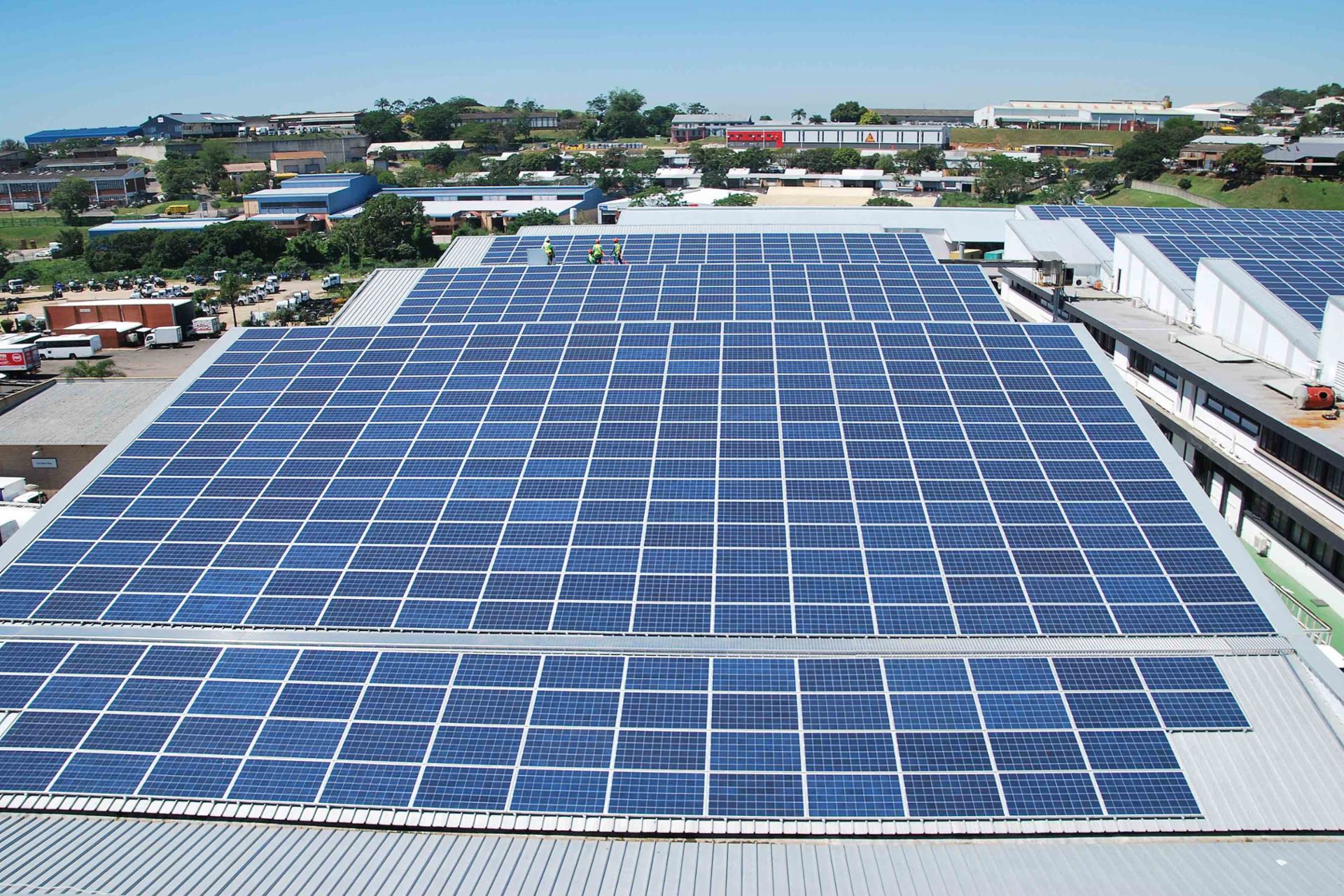
In a chemical process where carbon dioxide is combined with hydrogen, synthetic fuel is produced. This is known as e-fuels. Ideally, the electricity required for production comes from renewable sources, hence the “E” in e-fuels, signifying electric production technology. These synthetic, storable, and liquid energy sources serve as substitutes for diesel, petrol, or gas, boasting easy storage and transportation capabilities over extended periods.
Carbon dioxide for the process is sourced from the atmosphere, biomass, or industry. However, due to the exceptionally high energy demand of e-fuels in production, the process has faced criticism. Additionally, limited availability of green electricity, such as domestic wind power, in countries like Germany, makes production more feasible in countries with ample renewable energy sources. Presently, e-fuels are produced in small quantities, mainly in countries like Chile, and remain in the developmental stage. Despite generating pollutants like nitrogen oxides (NOx), particulate matter, and carbon monoxide during combustion, e-fuels are considered CO2-neutral overall, as the carbon dioxide released is equivalent to the amount consumed during production.
HVO – biogenic fuel made from plant waste
HVO 100 fuel, also known as HVO diesel, HVO fuel, or renewable diesel, represents a non-fossil diesel fuel crafted from renewable energy sources, organic materials, and residual oils, including used cooking oil. Beginning in spring 2024, HVO is to be sold in its pure form as HVO 100 at public petrol stations. Modern trucks and buses equipped with diesel engines can seamlessly operate using this biogenic fuel. However, discussions often highlight concerns regarding the potential misuse of food crops for fuel production, given that the current quantities of HVO available may not suffice to fuel all vehicles with this alternative fuel.
Many EU member states, such as Denmark, Belgium, the Netherlands and Sweden, as well as non-member states like Norway, have long authorized the use of HVO 100. In Germany, HVO was previously permitted only in blends of around 26% with fossil diesel. Notably, no additional carbon dioxide is emitted into the atmosphere when burning HVO 100, as only the CO2 previously absorbed by plants is released. Consequently, HVO is deemed CO2-neutral in terms of balance sheet accounting, although it is not entirely emission-free locally.
Expanding the range of biofuels: biodiesel, bioethanol, biomethane and biogas
Biodiesel stands out as another eco-friendly fuel option, compatible with conventional diesel engines and often viewed as a precursor to HVO. Widely recognized in Germany, biodiesel is blended with fossil diesel at levels of up to 7%, leading to reduced diesel consumption and related CO2 emissions. Notably, this renewable fuel is virtually sulfur-free. Its higher oxygen content enhances combustion, resulting in reduced engine residue and soot. Pure biodiesel, derived from rapeseed oil, remains functional even in temperatures as low as -12 degrees Celsius. In Germany, biodiesel production primarily relies on rapeseed oil. While biodiesel is considered CO2-neutral in terms of balance sheet accounting, it is not entirely emission-free locally.
Bioethanol serves as another globally utilized alternative fuel, commonly blended with petrol. In Germany, it makes up 5% or 10% of super or super plus petrol, denoted as E5 and E10, respectively. Also known as agricultural alcohol, bioethanol is produced exclusively from biomass. The starch present in biomass undergoes enzymatic breakdown into glucose, followed by fermentation with yeast to produce ethanol. Subsequently, distillation removes water from the resulting ethyl alcohol, achieving a purity level exceeding 99%. While bioethanol derived from plants offers advantages over fossil fuels in terms of CO2 emissions, the cultivation of these plants generates significant amounts of climate-damaging gases like nitrous oxide. Moreover, there is a contentious debate surrounding the environmental friendliness of ethanol production, particularly regarding the extensive land required for cultivation, often leading to monocultures.
Biomethane stands as a significant contributor to clean driving and climate protection and is obtainable from various sources. The most sustainable and cost-effective method approach involves utilizing local wastewater or organic waste. The use of certain types of waste, such as manure, offers a sustainable and circular solution that can lead to a CO2 reduction of over 100%. Scania, for example, offers trucks equipped with gas engines fueled by methane, leveraging the high density of liquefied natural gas (LNG) for efficient and eco-friendly transportation in regional and long-distance settings. Consequently, expanding the LNG infrastructure, including bio-LNG refueling stations, is crucial to meeting European climate protection goals, while promoting sustainable mobility in the commercial vehicle industry. Scania’s G-series offers high-performance vehicles tailored for climate-friendly mobility, catering to both LNG drive and CNG (compressed natural gas) propulsion systems.
Biogas shares molecular composition with natural gas (CNG), but unlike natural gas, it is a renewable fuel. Sourced regionally from renewable raw materials such as liquid manure, green waste, and renewable energy crops, biogas undergoes fermentation in specialized fermenters where these green materials decompose. Maize is the most efficient plant for biogas and is being increasingly cultivated. This has raised concerns from critics about the proliferation of maize monocultures and potential landscape degradation.
According to a recent study by the Stockholm Environmental Institute, biofuels will play a crucial role in reducing CO2 emissions from heavy goods transport in the coming decades. This will benefit both new and existing fleets. Over the past 25 years, the TRATON brand Scania has developed and marketed the largest range of engines capable of running on biofuels, such as biodiesel, HVO, and liquefied or compressed biogas. Recognizing that electrification alone cannot achieve the necessary CO2 reduction, Scania prioritizes sustainable biofuels as a cost-effective and CO2-efficient solution, often the only viable option for specific transport applications and markets.
Waiting for the future: initiating the market ramp-up for alternative fuels
One of the major challenges facing alternative fuels is their nationwide availability. Presently, the production of electricity-based fuels remains largely confined to demonstration and pilot plants. Even with the most optimistic projections, a significant market ramp-up seems unlikely in the foreseeable future, with forecasts not foreseeing substantial production volumes until after 2030. However, once the entire production chain is established and geared for industrial-scale production, the potential for generating synthetic natural gas alongside CO2-neutral diesel and petrol variants becomes feasible. These could serve as viable options for natural gas-powered internal combustion engines in trucks, among other applications.
The aviation industry is also actively exploring alternative fuels, aiming to transition to green paraffin at the earliest opportunity. Similarly to conventional combustion engine vehicles, aircraft have the potential to refuel CO2-neutral fuel. The EC has already mandated a blending obligation from 2025, meaning that aircraft departing from European airports must utilize alternative fuels. However, these regulations do not extend to road transport. In contrast, electrification is emerging as a logical and viable option for trucks and buses, offering a feasible pathway towards sustainability in the transport sector.